- Research & Instruments
- How we study - Our instruments and methods
- Instruments and Methods
- Methods
- Analysis of marine sugars
Analysis of marine sugars
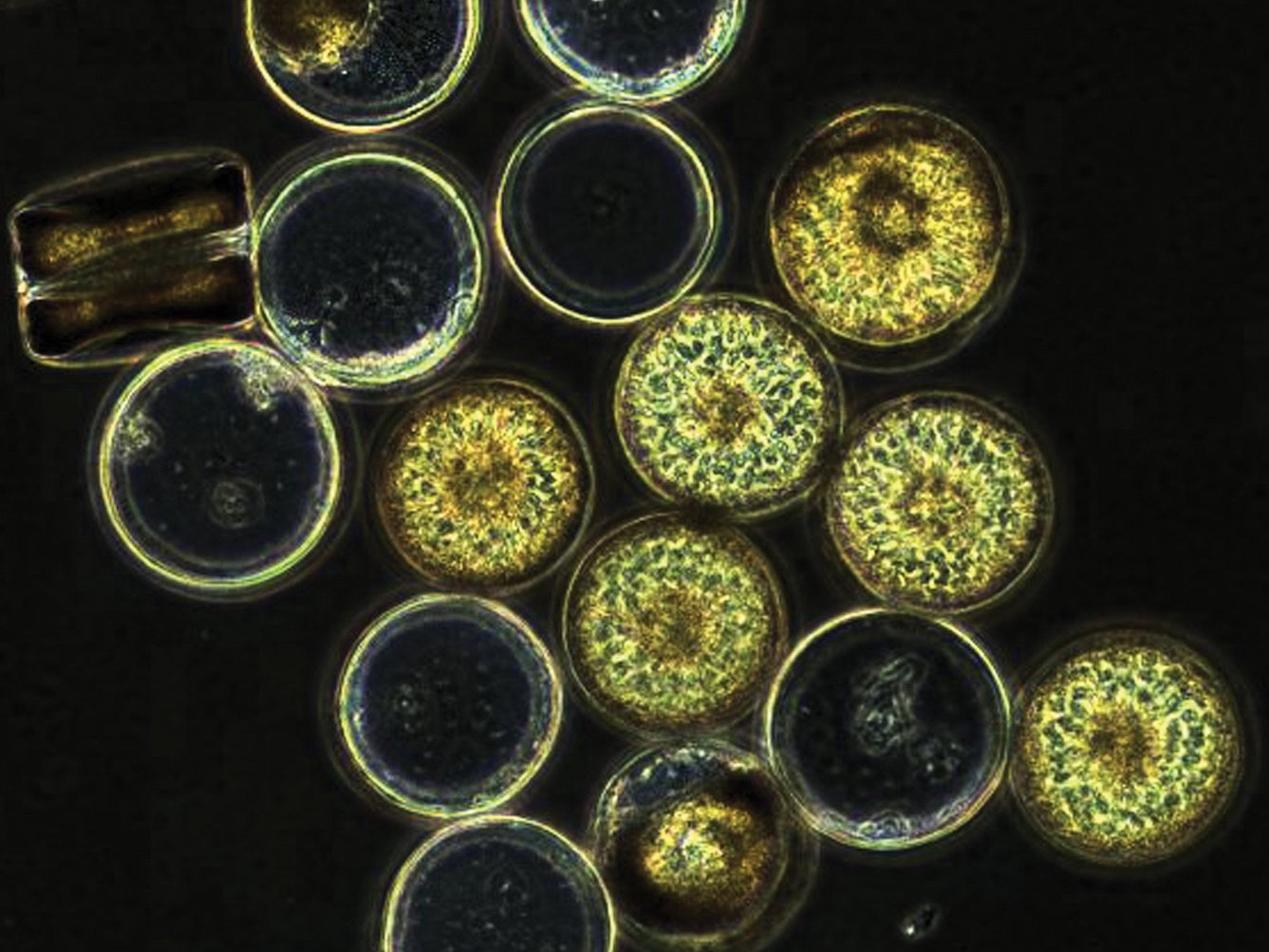
Why do we analyse sugars in marine research?
A major pathway for carbon sequestration in the ocean is the growth, aggregation and sinking of phytoplankton – unicellular microalgae like diatoms. Just like plants on land, phytoplankton sequester carbon from atmospheric carbon dioxide. When algae cells aggregate, they sink and take the sequestered carbon with them to the ocean floor. This so called biological carbon pump accounts for about 70 per cent of the annual global carbon export to the deep ocean. Estimated 25 to 40 per cent of carbon dioxide from fossil fuel burning emitted by humans may have been transported by this process from the atmosphere to depths below 1000 meter, where carbon can be stored for millennia.
Yet, even it is very important, it is still poorly understood how the carbon pump process works at the molecular level. Scientists of the research group Marine Glycobiology, which is located at the Max Planck Institute for Marine Microbiology and the MARUM – Center for Marine Environmental Sciences at the University of Bremen, investigate in this context marine polysaccharides – meaning compounds made of multiple sugar units – which are produced by microalgae. These marine sugars are very different on a structural level and belong to the most complex biomolecules found in nature. In the ocean microalgae produce a lot of sugar during algae blooms. These enormous quantities of algal biomass are normally recycled rapidly by marine bacteria. Especially sugars have been considered as easily digestible and therefore poor candidates for natural carbon sequestration. Now, new research results show: There exists a sugar in algae that resists rapid microbial degradation.
Thus – according to the hypothesis of the research group – polysaccharides could also be responsible for a more rapid movement of carbon-rich material in the ocean, much like the movement of food in humans. Therefore, they could help with the storage of carbon in the depths of the ocean and in the marine sediment.
How are algal sugars analysed?
These marine sugars are very different on a structural level and belong to the most complex biomolecules found in nature. One single bacterium is not capable to process this complex sugar-mix. Therefore a whole bunch of metabolic pathways and enzymes is needed. In nature, this is achieved by a community of different bacteria that work closely and very efficiently together – a perfect coordinated team.
In order to find microbially resistant sugars, the Bremen scientists have sought out a competent teacher: The sugar-degrading bacteria. These bacteria have found ways of finding polysaccharides and using them as food. They implement proteins to capture polysaccharides and enzymes to digest them. We are investigating how bacteria recognize polysaccharides and how their enzymes work. Every bacterium has its very own enzymes – its own tools – in order to cut polysaccharides. The bacterial enzymes are responsible for the breaking down of sugar compounds – they break the large, complex polysaccharides into small, simple monosaccharide units. These simple sugars are easier to measure than polysaccharides.
By measuring simple sugars, much like in a diabetes blood sugar test, it is possible to quantify polysaccharides. Thanks to this approach, it is now possible to measure which sugars are most common, which sink into the depths and thus act as a fiber in the ocean. This, in turn, allows conclusions to be drawn about the role of polysaccharides in the carbon cycle.
Which devices are needed for the analysis of marine sugars?
Because sugar analysis involves complex procedures, several different devices and techniques are used. Here we present the most important ones.
Algal cultivation
To analyse the sugars of microalgae, the algal bloom off the coast of Helgoland is regularly sampled. We also cultivate microalgae at the Institute in order to find out which species of algae produce which polysaccharides.
High performance liquid chromatography
High performance liquid chromatography (HPLC) plays an important role in the analysis of algal sugars. Liquid chromatography is a technique used to separate a mixture of substances based on interactions with the solid stationary phase and the mobile phase, which contains the liquid to be separated. The principle of this form of analysis or separation is explained here. In sugar analysis, either enzymes or hydrochloric acid are first used to break the marine polysaccharides into smaller fragments. These fragments can then be separated by HPLC based on their size and structure and identified with the respective standards.
This process uses the enzymes of sugar-eating bacteria, which are produced in large quantities in genetically modified bacteria. Once the exact functioning of the enzymes is determined using enzyme assays and X-ray structure analysis (see below), they can be used to break down the polysaccharides into monosaccharides, which are much easier to analyse. In contrast to hydrochloric acid, the enzymes allow very specific fragments to be produced. This procedure can be used to determine how the individual monosaccharides are linked (because enzymes can usually recognize and cleave only certain compounds) and in which proportions the different monosaccharides are present in the original sample.
Micro-Arrays
This method originally comes from medical and plant research. It combines the high throughput capacity of micro-arrays with the accuracy of monoclonal antibodies. The scientists first extract the sugar molecules from the seawater samples and then feed them into a machine that works a bit like a printer. This “printer” essentially “prints” the polysaccharides onto nitrocellulose paper, the micro-array. A micro-array is similar to a microchip; even though it is only the size of a fingernail, it can contain hundreds of samples. Monoclonal antibodies are added to the sugar molecules on the micro-array. Because the individual antibodies react with only one specific sugar, the researchers can see which monosaccharides are present in the sample.
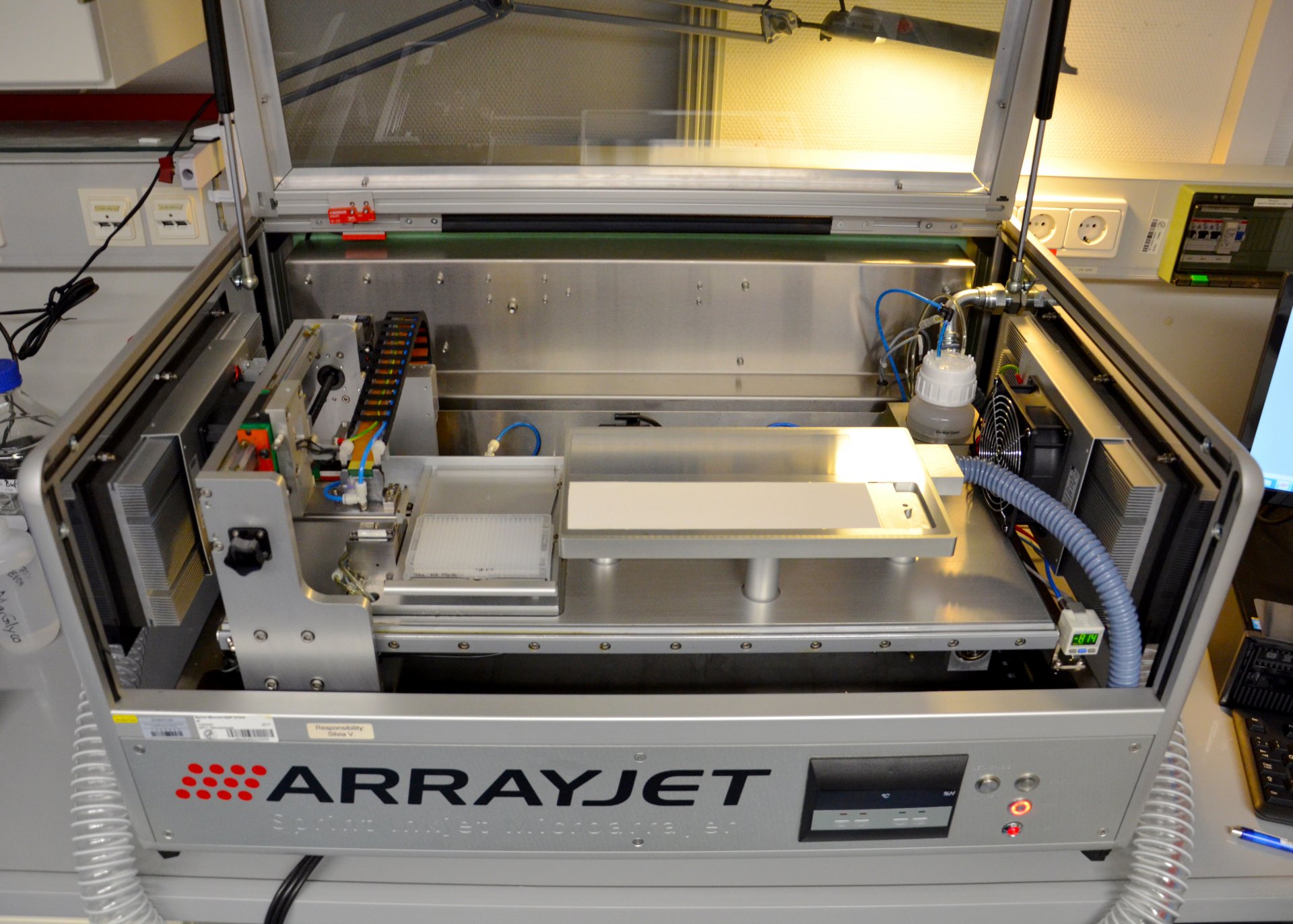
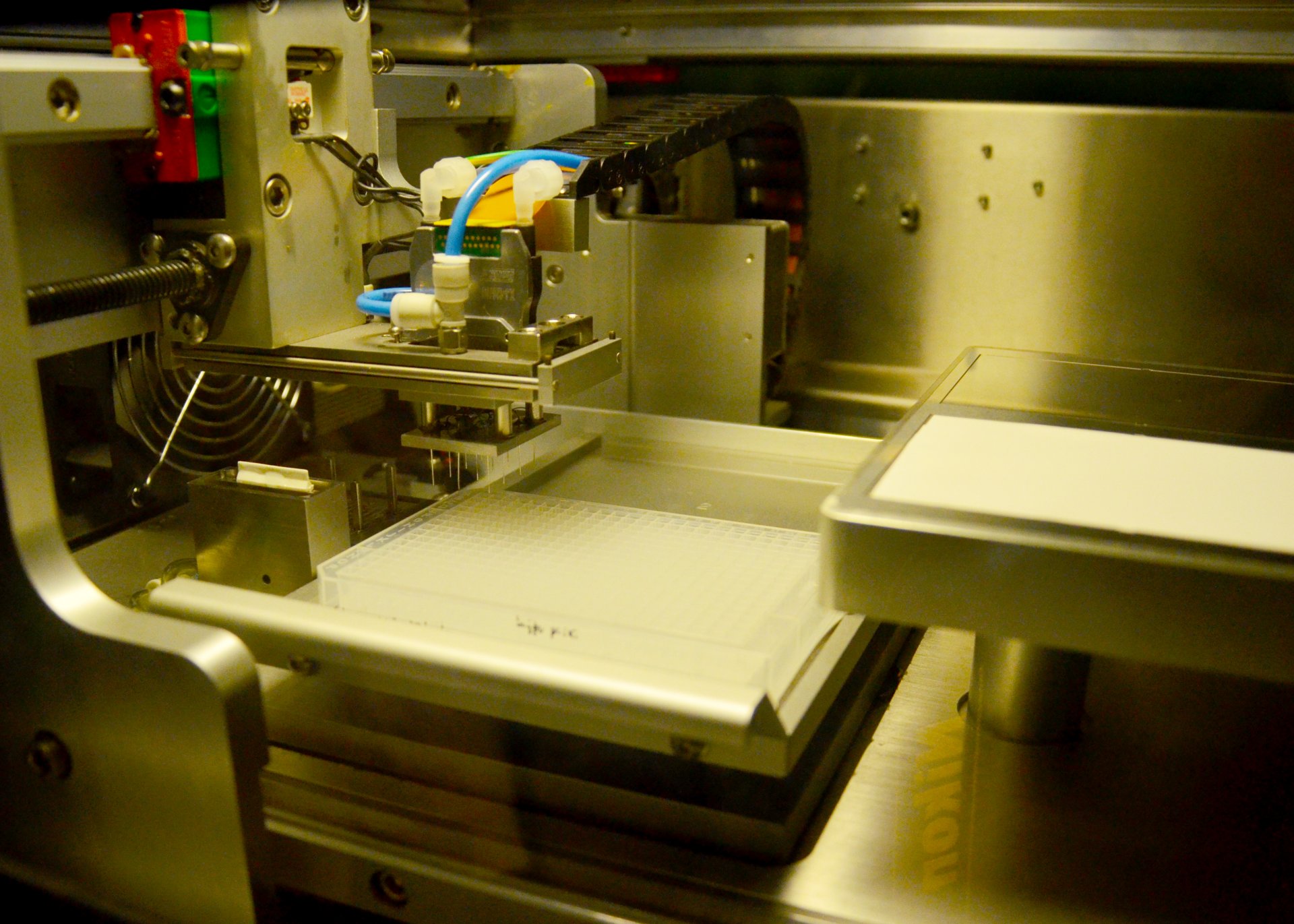
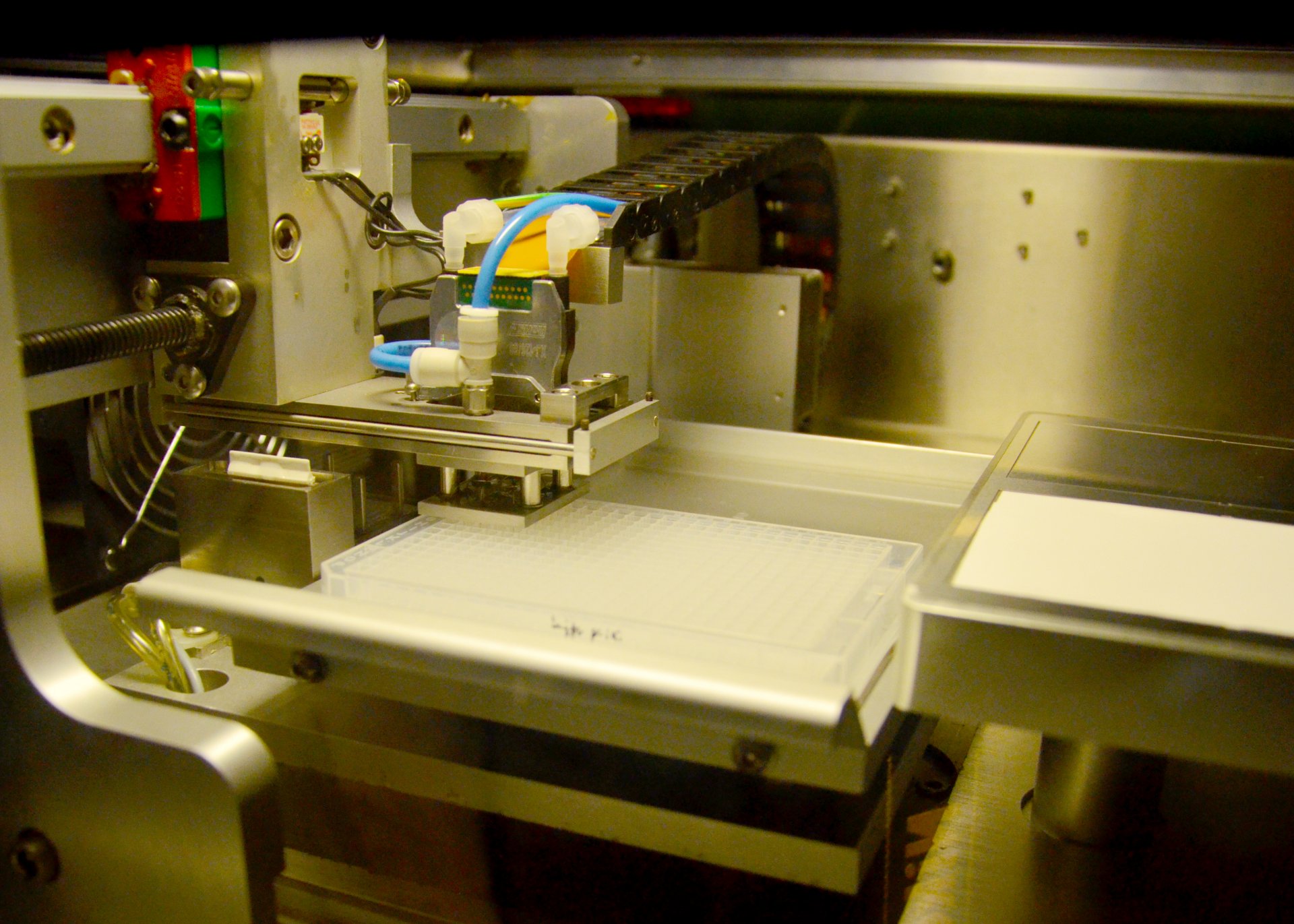
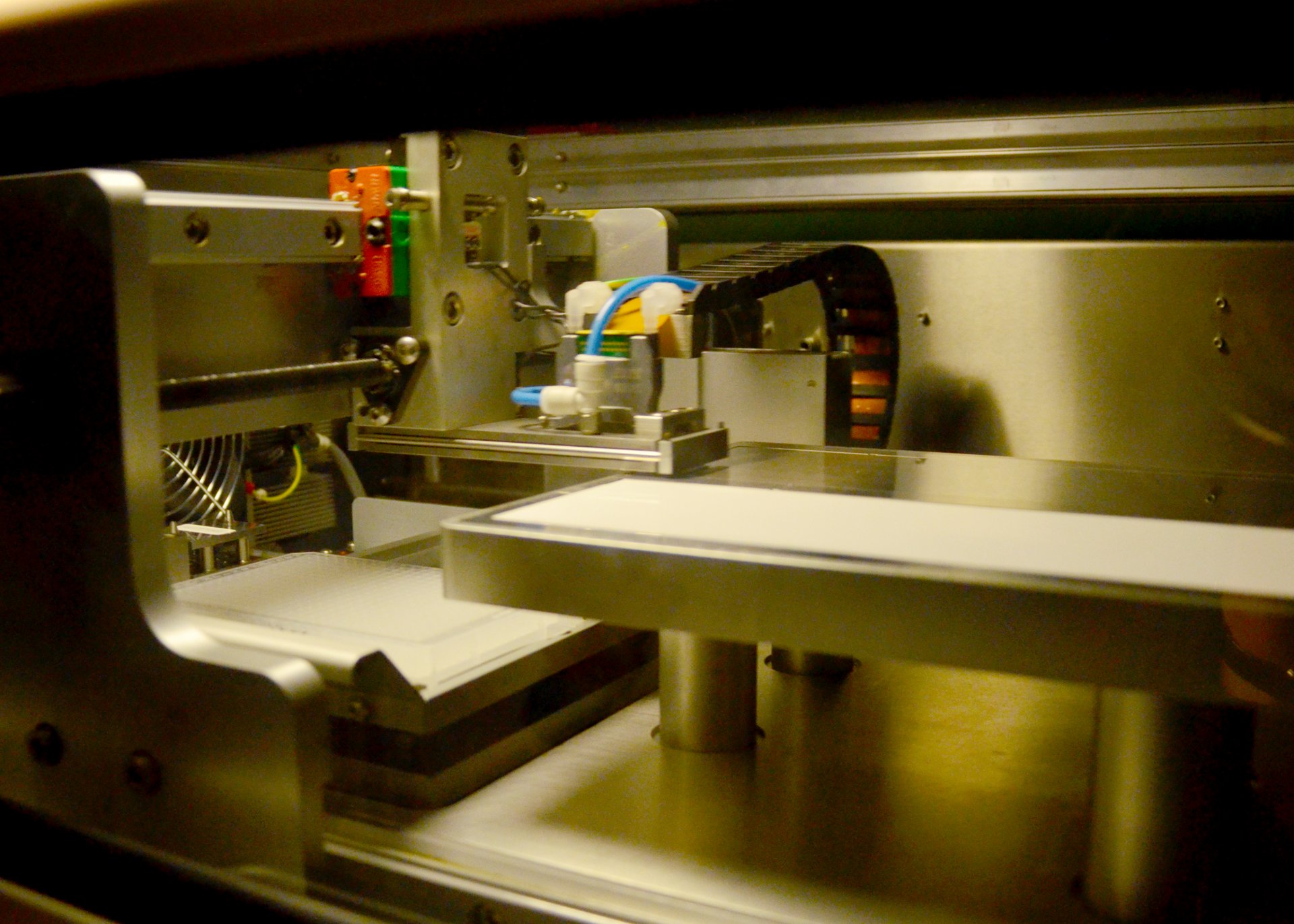
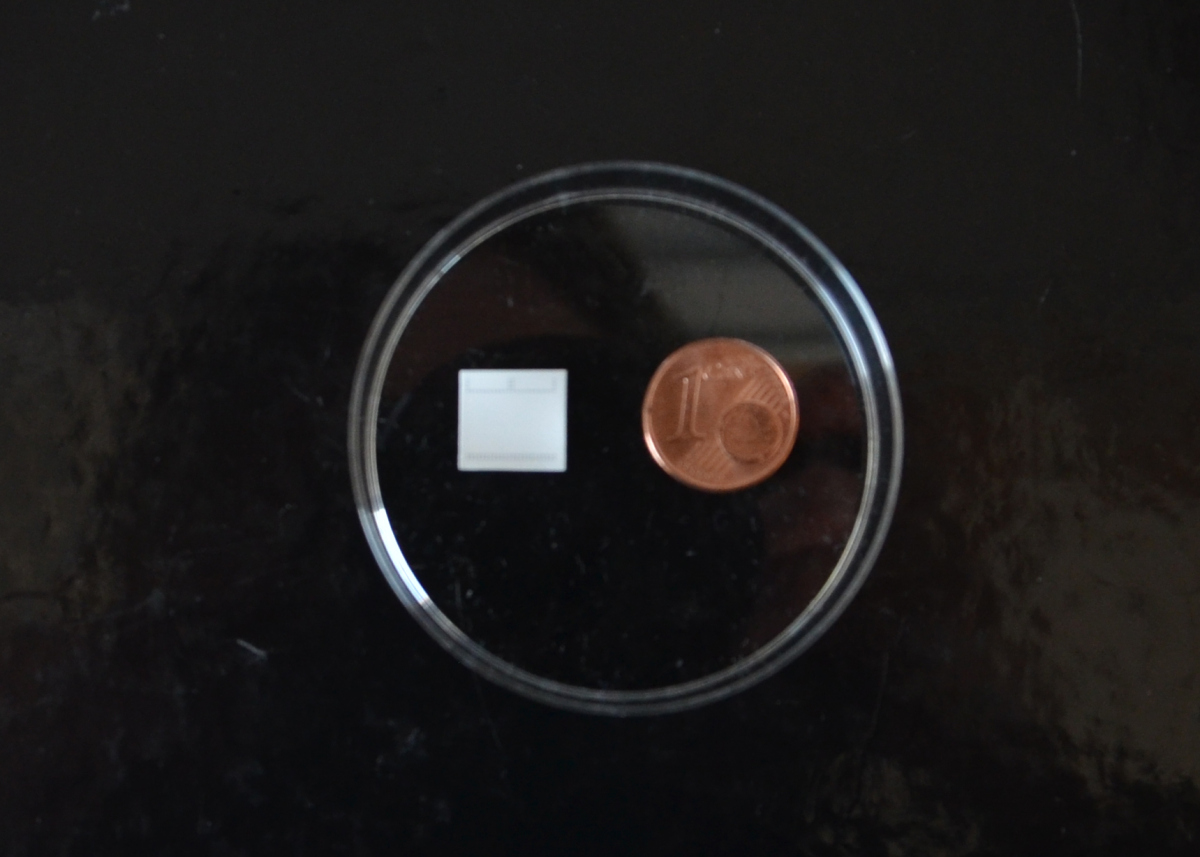
X-ray structure analysis
The enzymes produced in large quantities are extracted from the bacteria and separated using several liquid chromatography techniques (e.g. IEX, IMAC) until only minimal quantities of other enzymes and salts are present. The enzymes are then mixed with liquids that slowly strip the water from the enzymes, forcing them into a crystalline form. The crystals formed are then removed from the liquid, flash-frozen with liquid nitrogen, and sent to a partner laboratory, where they irradiated with X-rays. This method allows the scientists to look deep inside the enzymes and gain insights into the structure and function.
They can find not only enzymes that cut up the polysaccharides but also those that only bind and hold on to these polysaccharides and function similar to antibodies.
Example projects
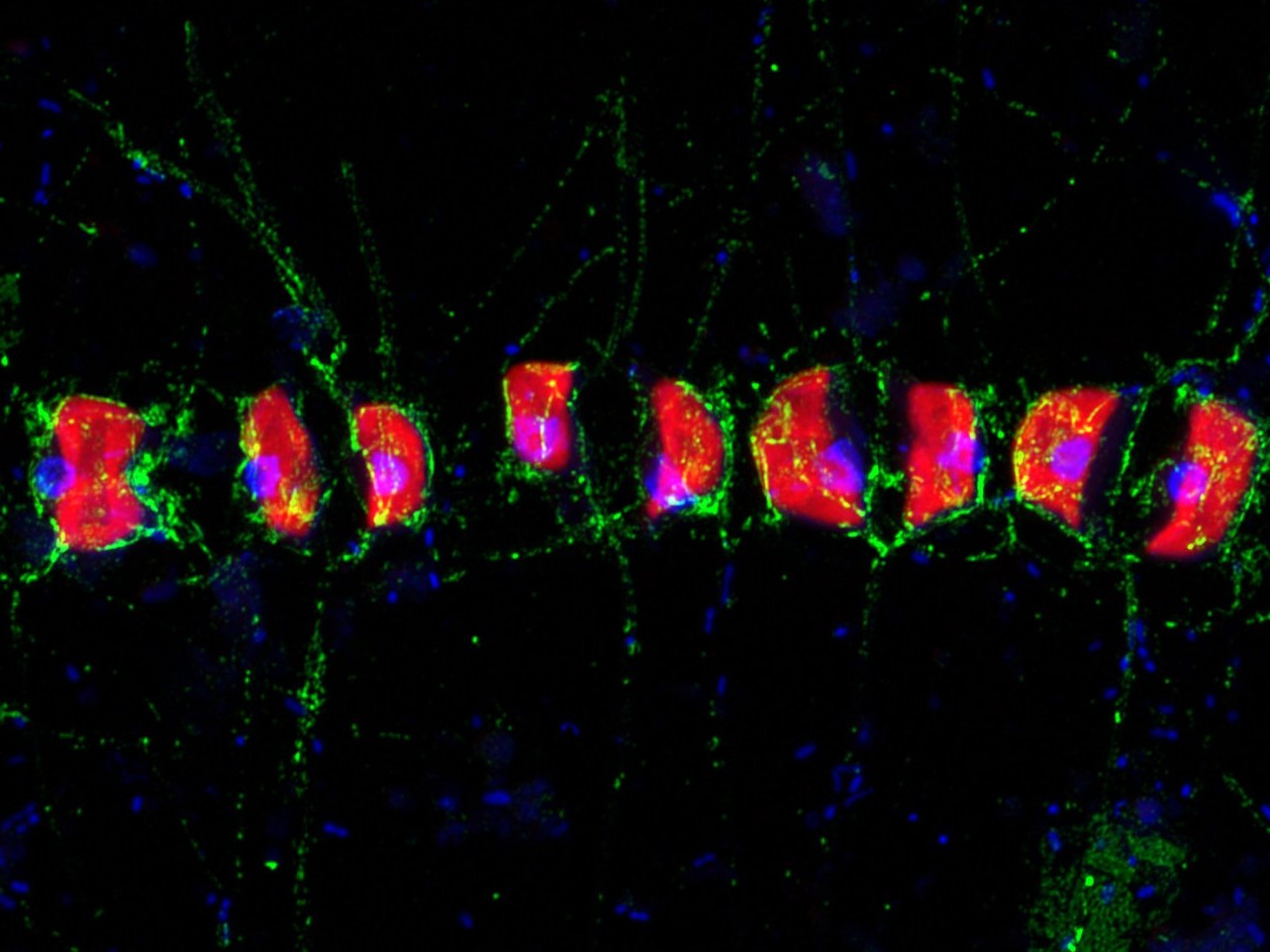
Sweet marine particles resist hungry bacteria
Rather sweet than salty: In the ocean microalgae produce a lot of sugar during algae blooms. These enormous quantities of algal biomass are normally recycled rapidly by marine bacteria – a degradation process that is an important part of the global carbon cycle. Especially sugars have been considered as easily digestible and therefore poor candidates for natural carbon sequestration. Now scientists from Bremen revealed: There exists a sugar in algae that resists rapid microbial degradation, accumulates, aggregates into particles and stores carbon during spring blooms. With this finding, published in the scientific journal Nature Communications, they show that this sugar can potentially act as an important carbon sink.
Read more in the press release "Sweet marine particles resist hungry bacteria"
Original publication:
Silvia Vidal-Melgosa, Andreas Sichert, T. Ben Francis, Daniel Bartosik, Jutta Niggemann, Antje Wichels, William G.T. Willats, Bernhard M. Fuchs, Hanno Teeling, Dörte Becher, Thomas Schweder, Rudolf Amann, Jan-Hendrik Hehemann: Diatom fucan polysaccharide precipitates carbon during algal blooms. Nature Communications, February 2021
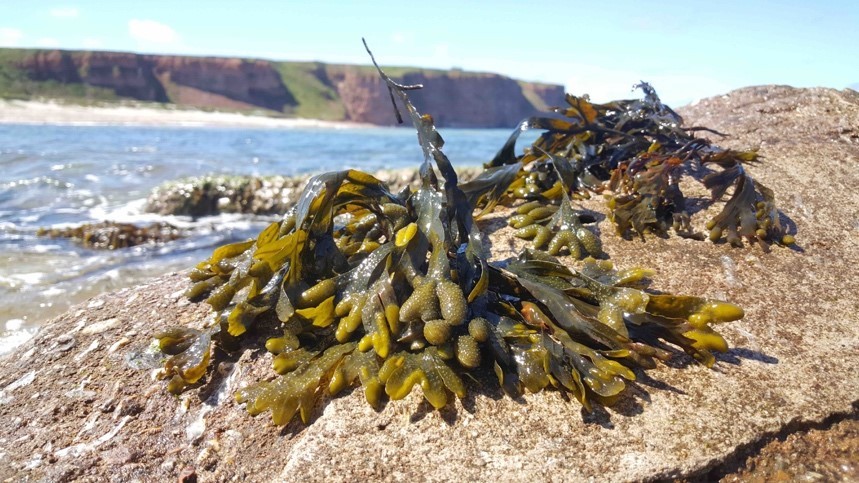
Sugar turns brown algae into good carbon stores
Brown algae are important players in the global carbon cycle by fixing large amounts of carbon dioxide and thus extracting this greenhouse gas from the atmosphere. Moreover, because microbial decomposition of dead brown algae is slower than that of other marine plants, carbon dioxide fixed by brown algae remains much longer in the sea. Scientists from the Max Planck Institute for Marine Microbiology, the MARUM – Center for Marine Environmental Sciences at the University of Bremen and other institutes therefore explored why brown algae degrade so slowly. They found that only highly specialized bacteria can carry out the degradation with the help of more than hundred enzymes.
Read more in the press release "Sugar turns brown algae into good carbon stores"
Original Publication:
Andreas Sichert#, Christopher H. Corzett#, Matthew S. Schechter, Frank Unfried, Stephanie Markert, Dörte Becher, Antonio Fernandez-Guerra, Manuel Liebeke, Thomas Schweder, Martin F. Polz, Jan-Hendrik Hehemann: Verrucomicrobia use hundreds of enzymes to digest the algal polysaccharide fucoidan. Nature Microbiology, May 2020
DOI: 10.1038/s41564-020-0720-2
# both authors contributed equally
Contact
Group leader
MARUM MPG Bridge Group Marine Glycobiology
MPI for Marine Microbiology
Celsiusstr. 1
D-28359 Bremen
Germany
Room: |
2126 |
Phone: |
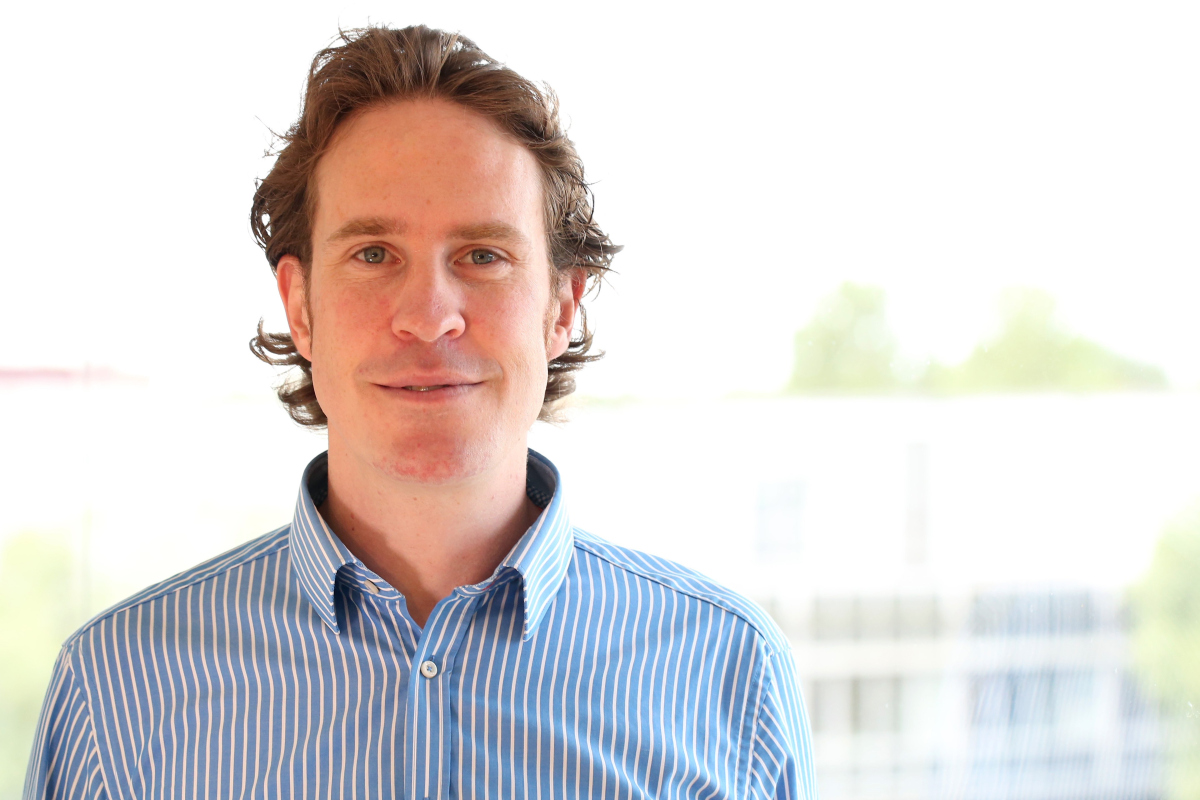