Chemostat
What is continuous cultivation?
Most microorganisms live under substrate limitation, as the supply of their substrates depends on other organisms or physicochemical properties of their environment. In conventional microbiological approaches one or more substrate(s) are supplied to samples in excess, as a result the fastest growing species is brought to culture. This does not reflect what happens in the environment. In the Microbial Physiology group, we use continuous cultivation bioreactors, which closely mimic the environments that microorganisms live in – in the laboratory, under controlled conditions. That allows us to culture environmentally-relevant microorganisms to study their physiological and biochemical properties in molecular detail.
In our laboratory, we use chemostats and other types of continuous cultivation techniques. In a chemostat, fresh medium is continuously added to the culture vessel while a corresponding volume of culture liquid is removed at the same rate.
How does a chemostat work?
A chemostat usually consists of a closed (glass) vessel with a volume between 1 ml and a few liters. Fresh medium is supplied continuously with an influent pump. A second pump removes liquid from the vessel at the same rate. This way a constant volume is maintained. The microorganisms added to the vessel can only consume what is supplied by the influent pump. Their growth rate (per hour) is defined by the ratio of the influent rate (liter or milliliters per hour) and the vessel volume (L). This state can be maintained indefinitely. Among the substrates and growth factors added to the medium, one is the so-called controlling substrate, which limits growth.
The others are present in surplus. The concentration of the controlling substrate determines the concentration of cells in the vessel and in the effluent. In most cases the energy or carbon source serves as the controlling substrate, but it can be adjusted depending on your research question. Furthermore, parameters such as pH, temperature and mixing can be easily and accurately controlled and manipulated.
Chemostats in action
A chemostat is an excellent tool to cultivate microorganisms, as the conditions are defined and constant compared to batch cultures (e.g., shake flasks). This makes experiments easier to reproduce. Furthermore, the set-up generates a continuous flow of microbial culture, much like your tap generates a continuous flow of clean water, that can be conveniently harvested for further experiments. The chemostat can be used for pure cultures to study the kinetics of microbial growth, as well as for more detailed -omics approaches. It can also be used for competition experiments. In such experiments two or three different microorganisms with comparable niches are released in the vessel under varying conditions, at high or low growth rates, at high or low oxygen concentrations, distinct pH or temperature values, with or without growth factors, etc.
There are different kinds of chemostat setups, allowing for different research questions to be posed and different organisms to be targeted. For example, some microorganisms might prefer to grow as biofilm or biofilm aggregates. They can be catered to e.g. by introducing more surface area to the bioreactor for microorganisms to attach or by adding a settler unit. Slow-growing microorganisms can be retained in chemostats using membrane filters that keep the microorganisms in the bioreactor, while letting spent medium through (so-called retentostats). In order to grow microorganisms directly from sandy sediments, we can use cylindrical cores equipped with precise pump units. Here, part of the control over the enrichment culture is lost as microniches can form throughout the (sandy) sediment core.
Example projects
Greenhouse Gas Mitigation through Advanced Nitrogen Removal Technology - GREENT
Human activities have severe impacts on the biological carbon and nitrogen cycles, the most important consequences of these are global warming and water pollution. Wastewater treatment technology, in particular nitrogen removal systems, improved considerably in the last decade. The application of anaerobic ammonium oxidizing (anammox) bacteria in oxygen-limited granules has the potential to turn wastewater treatment plants into energy-efficient systems with minimal greenhouse gas emissions. Recently, microorganisms that couple the anaerobic oxidation of methane to denitrification were discovered. An innovative integration of these microorganisms into certain systems for wastewater treatment offers an elegant and efficient solution to combat greenhouse gas emissions from wastewater treatment plants. The aim of the GREENT project is to determine nitrous oxide emissions from partial nitritation-anammox bioreactors and the parameters that govern these emissions, and to investigate the responsible pathways in molecular detail. Furthermore, it explores the feasibility of an innovative bioreactor, which will remove ammonium and methane simultaneously through anammox and anaerobic methane-oxidizing microorganisms.
More Information: https://www.mpi-bremen.de/ERC-Project-GREENT.html
Microbe hunters discover long-sought-after iron-munching microbe
A microbe that ‘eats’ both methane and iron: microbiologists have long suspected its existence, but were not able to find it. In 2016, researchers at Radboud University and the Max Planck Institute for Marine Microbiology in Bremen discovered a microorganism that couples the reduction of iron to methane oxidation, and could thus be relevant in controlling greenhouse gas emissions worldwide.
Here you can find the press release: https://www.mpi-bremen.de/en/Microbe-hunters-discover-long-sought-after-iron-munching-microbe.html
Microbes can grow on nitric oxide (NO)
Nitric oxide (NO) is a central molecule of the global nitrogen cycle. A study by Boran Kartal and colleagues reveals that microorganisms can grow on NO at concentrations that would be lethal to all other lifeforms. Their results, which were published in Nature Communications, change our view of the earth’s nitrogen cycle and how microorganisms regulate the release of greenhouse gases from natural and man-made environments.
Here you can find the press release: https://www.mpi-bremen.de/en/Microbes-can-grow-on-nitric-oxide-NO.html
Discovery of an unusual protein
Scientists from Bremen discover an unusual protein playing a significant role in the Earth’s nitrogen cycle. The novel heme-containing cytochrome is involved in the anammox process, which is responsible for producing half of the dinitrogen gas in the atmosphere and important in greenhouse gas regulation.
Here you can find the press release: https://www.mpi-bremen.de/en/Discovery-of-an-unusual-protein.html
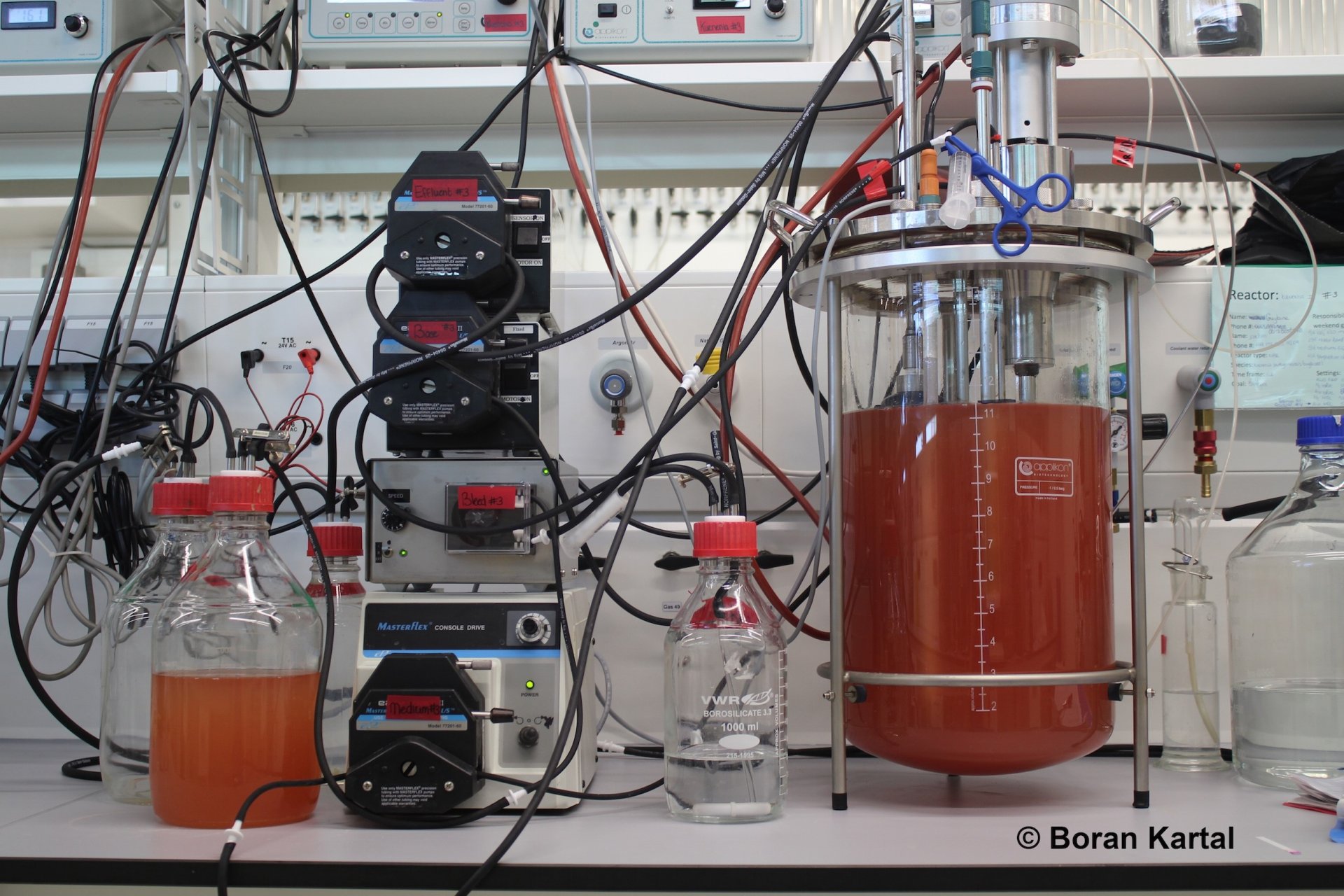
Please direct your queries to
Group leader
Microbial Physiology Research Group
MPI for Marine Microbiology
Celsiusstr. 1
D-28359 Bremen
Germany
Room: |
3126 |
Phone: |
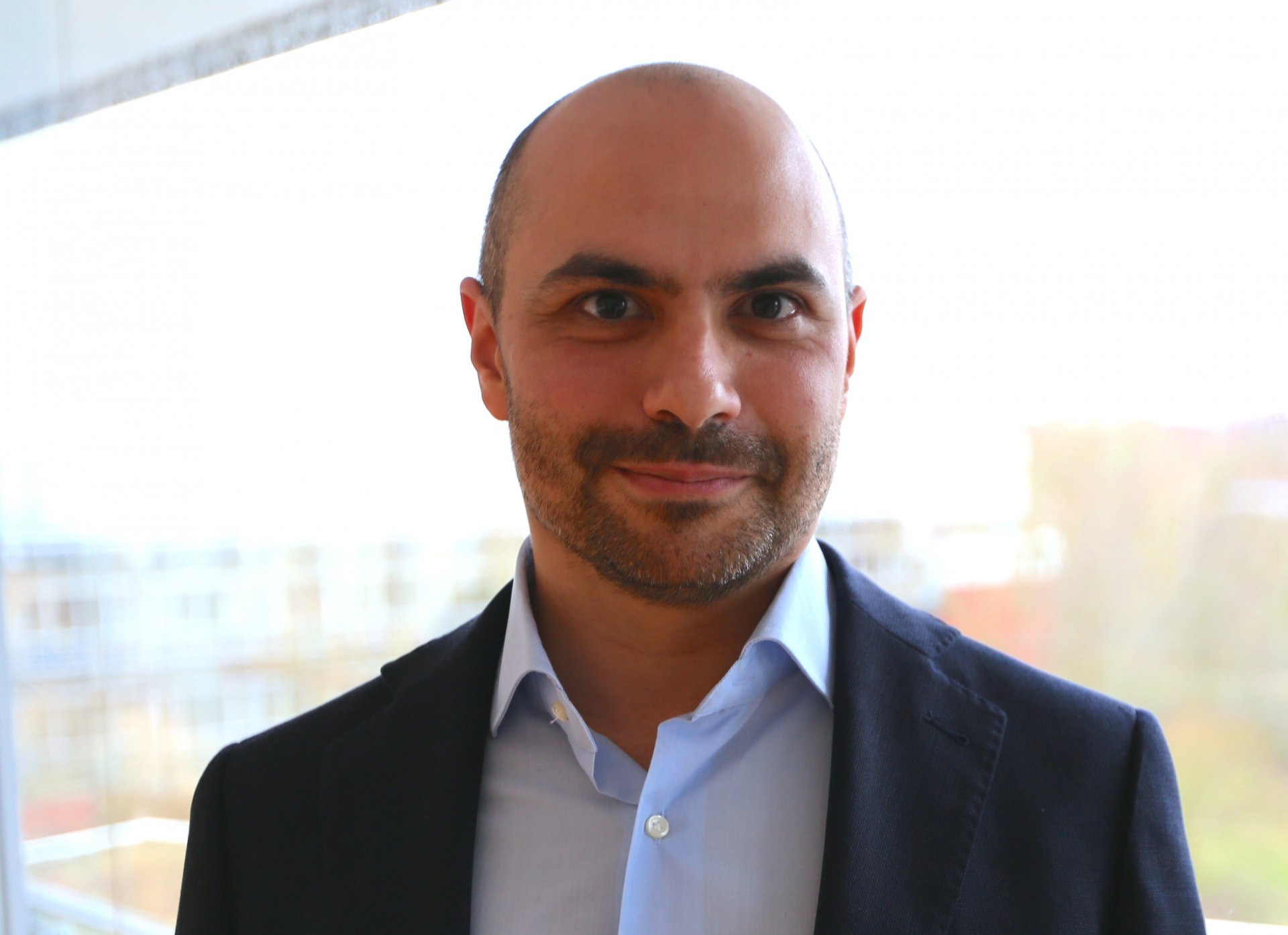