Seitenpfad:
- Presse
- Pressemeldungen 2009
- 31.08.2009 LOOME: Langzeit-Observatorium gestartet
31.08.2009 LOOME: Langzeit-Observatorium gestartet
LOOME
Long-term Observatory On Mud-volcano Eruptions
A demonstration mission to establish a long term observatory on a methane emitting deep-sea mud volcano.
LOOME coordinator:
Dirk de Beer, +49 421 2028 802 or [Bitte aktivieren Sie Javascript]
Max-Planck-Institute for Marine Microbiology, Celsiusstrasse 1, 28359 Bremen, Germany
Principal Investigators and their institutions
Long-term Observatory On Mud-volcano Eruptions
A demonstration mission to establish a long term observatory on a methane emitting deep-sea mud volcano.
LOOME coordinator:
Dirk de Beer, +49 421 2028 802 or [Bitte aktivieren Sie Javascript]
Max-Planck-Institute for Marine Microbiology, Celsiusstrasse 1, 28359 Bremen, Germany
Principal Investigators and their institutions
Institution | Principal Investigator | Address | |
Max Planck Institute for Marine Microbiology (KDM partner) | Dirk de Beer | Celsiusstrasse 1, D-28359 Bremen [Bitte aktivieren Sie Javascript] Tel. 0421 2028– 802 Fax: 0421 2028- 690 | Germany |
MARUM (KDM partner) | Christoph Waldmann | Leobener Str. D-28359 Bremen [Bitte aktivieren Sie Javascript] Tel. 0421 218 – 65606 Fax: 0421 218 – 65605 | Germany |
AWI (KDM partner) | Michael Schlueter | Am Handelshafen 12, 27570 Bremerhaven, Germany [Bitte aktivieren Sie Javascript] Tel .+49 471 4831 1840 Fax:+49 471 4831 1425 | Germany |
IfM Geomar (KDM partner) | Thomas Feseker | Wischhofstr. 1-3, 24148 Kiel, Germany [Bitte aktivieren Sie Javascript] Tel. +49 431 6002321 Fax: +49 431 6002916 | Germany |
Ifremer | Jean Paul Foucher | Technopole de Brest-Iroise BP 70 29280 PLOUZANE, France [Bitte aktivieren Sie Javascript] Tel: +33 (0)2 98 22 40 40 FAX: +33 (0)2 98 22 40 45 | France |
UiT | Juergen Mienert | Dramsveien 201 N-9037 Tromsø, Norway [Bitte aktivieren Sie Javascript] Telephone: (+47) 77 64 44 46 Fax: (+47) 77 64 56 00 | Norway |
Introduction
LOOME DM is a networking action for the long-term observation of a major site of methane emission from the deep European margin, the Håkon Mosby mud volcano (HMMV). The HMMV is a cold seep ecosystem located at a water depth of 1250 m on the SW Barents Sea slope off Norway, in an area with a history of seabed slides and tsunamis, and under exploitation for hydrocarbon resources and fisheries. The Barents Sea slope is a target area for sustainable management and monitoring of global change effects. Previous work of the partners at HMMV yielded evidence of several eruptive events, indicated by strong gas ebullition and abrupt temperature changes of almost 10°C within a few days. High-resolution bathymetric maps and video observations of the seafloor before and after this event clearly showed changes in the morphology of HMMV. Only by detailed continuous observations, recording a wide variety of parameters, we can learn about the mechanics of such eruptions and their early signals, estimate the amount of gas released and the consequences for
geochemistry and local communities as well as for seafloor stability, which are main scientific objectives.
Furthermore, methane is a powerful greenhouse gas and therefore the global budgeting of sources and sinks is of great importance. Searches for marine sources of methane are focused on deep-sea seepage through mud volcanoes and gas hydrate bearing sediments. It is thought that in these areas most methane transported towards the oxic biosphere is removed by anaerobic methane oxidation coupled to sulfate reduction (1-3, 5, 6). The efficiency of methane transport and oxidation in the seafloor are, however, still poorly constrained due to lack of understanding of the controlling factors. Special conditions, for example by high pore water flow or gas ebullition by excessive methane accumulation, enable the methane to escape up through the sulfate barrier (4).
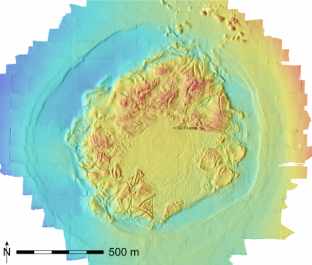
Microbathymetric map from 2003. (Source: IFREMER) The volcano is surrounded by a slight depression.
Ecology and transport
Liquefied mud, gas, and geofluids rising from a depth of at least three kilometers, form a highly active mud volcano withpermanent gas emission. The Haakon Mosby is ca 1200 m in diameter, has max 10 m height and consists of several more or less concentric habitats. It is a site of unique chemoautrophically driven faunal and microbial communities, fuelled by the oxidation of methane by sulfate and further oxydation of sulfide by oxygen and nitrate. An outer hummocky area of ca 300 m width is shaped by gas hydrates, and covered with Pogonophora worms. Gray bacterial mats occur above highly gassy sediment patches where hydrates have eroded. Further towards the center one finds large mats of Beggiatoa, large filamentous bacteria that oxidise sulfide with oxygen or nitrate. The center is flat and consists of gray mud. Here the highest flow velocities occur, that gradually decrease outwards, and are probably close to zero in the hydrate zone. This ecological structure can be understood from the differences in porewater seepage(2).
The higher flow velocities in the center are reflected in the higher surface temperatures, and more specifically, in steeper near surface temperature gradients. A detailed study using temperature probes, showed that the steepest gradients, and thus the highest upflow velocities, are present in an area of ca 80 m in diameter near the north side of the central area, characterized by highly disturbed, gas saturated sediments (Fig. Hot spot area).
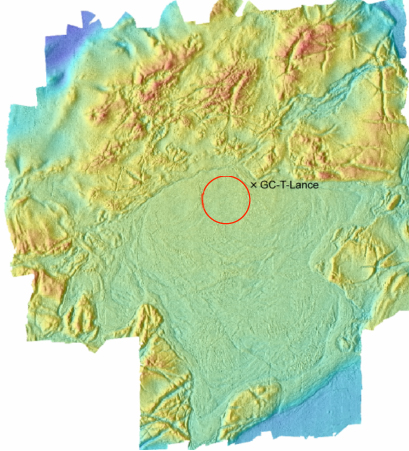
Microbathymetric map from 2006 (Source IFREMER), with a 10 times higher resolution. The hot center is indicated with a red circle. The X indicates the position of the graviticorer with attached temperature probes.
We obtained evidence from a year long temperature record, that the HMMV is not in a continuously seeping vent. In December 2005 and April 2006 drastic temperature changes were observed, occurring in one day. These spectacular phenomena show that the center of the HMMV is active and has irregular eruptions. The disturbance caused by these eruptions will affect biogeochemistry and local fauna. Thus the ecological zones can be understood from a steady state model and from temporal local perturbations. To assess which phenomena are the dominant shaping factors is fascinating from fundamental scientific point of view.
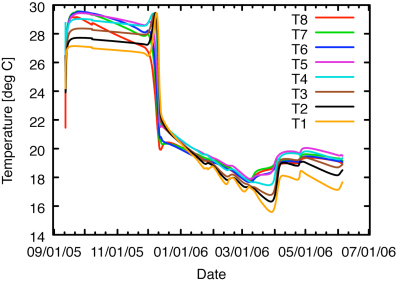
The site forms thus a natural laboratory for ecologists and geologists. It was selected to be included in the Norwegian Margin cable network. The HMMV is a priority target within the ESONET/EMSO project, and a key site of the EU projects HERMES/HERMIONE, MARBEF as well as the ESF EuroDeep program CHEMECO.
The observatory
We proposed LOOME as demonstration mission within ESONET, to deploy a long term observatory with a large variety of sensors to prepare for a node connected measuring system. In 2008 we were granted k€300 as encouraging support for this plan, totaling k€1300. The scientific aims of LOOME are to document physico-chemical phenomena before, during and after an eruption, and to study its effects on gas hydrate stability, seafloor morphology, geochemistry and the distribution and colonization patterns of benthic communities.. The technological aims are to integrate many sensors and to define best parameters for further long term observation of mud volcanism, to optimize integrated ways of underwater data storage and retrieval, and to develop technology for wake up calls.
We aimed for a combination of sensors that measure phenomena deep into the volcano, at the surface and in the water column. Moreover, as the system must be autonomous, the boundary conditions for the choice of sensors were determined by data storage and power consumption. Most essential is that a possible eruption would not result in data loss, therefore all data measured at the eruption site will be stored in the frame of the LOOME observatory on a safe adjacent location 15 m away from the hot spot
First Installations in 2008
In autumn 2008 the first autonomous moorings were deployed by a joint mission of the University of Tromso and IFREMER with RV Jan Mayen (Norway). The moorings included an OBS system, a temperature lance and a piezometer. These moorings were recovered during our recent expedition with POLARSTERN (ARK XXIV-2, 10.07.09 – 04.07.09) and redeployed
The observatory
We proposed LOOME as demonstration mission within ESONET, to deploy a long term observatory with a large variety of sensors to prepare for a node connected measuring system. In 2008 we were granted k€300 as encouraging support for this plan, totaling k€1300. The scientific aims of LOOME are to document physico-chemical phenomena before, during and after an eruption, and to study its effects on gas hydrate stability, seafloor morphology, geochemistry and the distribution and colonization patterns of benthic communities.. The technological aims are to integrate many sensors and to define best parameters for further long term observation of mud volcanism, to optimize integrated ways of underwater data storage and retrieval, and to develop technology for wake up calls.
We aimed for a combination of sensors that measure phenomena deep into the volcano, at the surface and in the water column. Moreover, as the system must be autonomous, the boundary conditions for the choice of sensors were determined by data storage and power consumption. Most essential is that a possible eruption would not result in data loss, therefore all data measured at the eruption site will be stored in the frame of the LOOME observatory on a safe adjacent location 15 m away from the hot spot
First Installations in 2008
In autumn 2008 the first autonomous moorings were deployed by a joint mission of the University of Tromso and IFREMER with RV Jan Mayen (Norway). The moorings included an OBS system, a temperature lance and a piezometer. These moorings were recovered during our recent expedition with POLARSTERN (ARK XXIV-2, 10.07.09 – 04.07.09) and redeployed
The frame of LOOME is constructed of light weight non-corrosive and flexible materials. It can be recovered autonomously or by ROV. Summarizing the LOOME observatory measuring from depth to watercolumn:
The sensors measuring in depth are a Ocean Bottom Seismometer (OBS), a 8 m piezometer, and a T-lance of 13 m. 1) The OBS measures acoustics from the seafloor. The sounds from the seafloor are corrected with the sounds from the water column to obtain a clear image of the geosounds indicating seismic activity of the mud volcano. The data should give an early warning of an eruptive event. 2) The piezometer measures subtle changes in porewater pressure, thus indicative for changed porewater flow. 3) The T-lance will measure changes in the temperature profile, induced by changed porewater movement.
At the seafloor we will measure with a series of chemosensors and T-strings across the hot spot, and a 1 m T-lance in the middle of the hot spot. 1) The chemosensors are 6 units each measuring pH, DO and ORP. We hope to detect increased upward flow of the acidic, anoxic and low ORP porewater. 2) The T-string includes 24 thermometers, laid out across the hot spot. With these strings we hope to obtain a picture on the dynamics of surface phenomena.
In the water column we measure with three CTDs (conductivity, T, salinity, turbidity) mounted at the bottom, middle and top of the frame of LOOME, and a scanning sonar. 1) With the two CTDs we hope to detect the vertical extent of the effect of the seismic activity of the active site. 2) With the sonar we will detect gas flares up to a distance of 300 m, and can quantify the emissions to a distance of 50 m. The power consumption of the sonar and the data storage capacities do not allow the sonar to operate at sufficient intensity during 1 year, thus the sonar will switch on and off automatically.
As a first step towards the integration of these various sensors into an observatory, some of them are connected to a COSTOF (Communication and Storage Front-end), a low-power consumption modular electronic unit that provides the following services: synchronization of the measurement data with a common clock, local duplication of some sensor data and a common access channel to all connected sensors via a CLSI (Contact-Less Serial Interface) allowing the installing ROV to fine-tune and check the functioning of the sensors on the seabed. First, the COSTOF was planned to be used to trigger the activation of the sonar for 24 hours when an eruption is predicted. This is done by running an algorithm that analyses the T-lance data as and when acquired, to predict the occurrence of an eruption. The prediction algorithm was developed on the base of past temperature data series analysis. Unfortunately, the sonar crashed, could be repaired, but can no longer communicate with COSTOF, and is now programmed to record autonomously 23 scans every 12 hours. We expect still interesting data.
The sensors measuring in depth are a Ocean Bottom Seismometer (OBS), a 8 m piezometer, and a T-lance of 13 m. 1) The OBS measures acoustics from the seafloor. The sounds from the seafloor are corrected with the sounds from the water column to obtain a clear image of the geosounds indicating seismic activity of the mud volcano. The data should give an early warning of an eruptive event. 2) The piezometer measures subtle changes in porewater pressure, thus indicative for changed porewater flow. 3) The T-lance will measure changes in the temperature profile, induced by changed porewater movement.
At the seafloor we will measure with a series of chemosensors and T-strings across the hot spot, and a 1 m T-lance in the middle of the hot spot. 1) The chemosensors are 6 units each measuring pH, DO and ORP. We hope to detect increased upward flow of the acidic, anoxic and low ORP porewater. 2) The T-string includes 24 thermometers, laid out across the hot spot. With these strings we hope to obtain a picture on the dynamics of surface phenomena.
In the water column we measure with three CTDs (conductivity, T, salinity, turbidity) mounted at the bottom, middle and top of the frame of LOOME, and a scanning sonar. 1) With the two CTDs we hope to detect the vertical extent of the effect of the seismic activity of the active site. 2) With the sonar we will detect gas flares up to a distance of 300 m, and can quantify the emissions to a distance of 50 m. The power consumption of the sonar and the data storage capacities do not allow the sonar to operate at sufficient intensity during 1 year, thus the sonar will switch on and off automatically.
As a first step towards the integration of these various sensors into an observatory, some of them are connected to a COSTOF (Communication and Storage Front-end), a low-power consumption modular electronic unit that provides the following services: synchronization of the measurement data with a common clock, local duplication of some sensor data and a common access channel to all connected sensors via a CLSI (Contact-Less Serial Interface) allowing the installing ROV to fine-tune and check the functioning of the sensors on the seabed. First, the COSTOF was planned to be used to trigger the activation of the sonar for 24 hours when an eruption is predicted. This is done by running an algorithm that analyses the T-lance data as and when acquired, to predict the occurrence of an eruption. The prediction algorithm was developed on the base of past temperature data series analysis. Unfortunately, the sonar crashed, could be repaired, but can no longer communicate with COSTOF, and is now programmed to record autonomously 23 scans every 12 hours. We expect still interesting data.
Finally, 10 m away from the LOOME frame we positioned an autonomous camera that will at regular intervals takes video streams of gas bubble emissions from the fresh mud flow and the local fauna browsing bacterial mats.
LOOME just before deployment, left panel, Backside with central data storage, CLSI (funnel) and Costof, right photo: front side with T and chemosensor strings, sonar and CTD. The white blocks are flotation material.
Deployment and recovery
Deployment took place during the ARK-XXIV/2 FS Polarstern cruise (cruise leader M. Klages) in July 2009 using the ROV QUEST4000 of MARUM. LOOME was lowered to the seafloor by winch, and accurately positioned by the ROV Quest after the release of its deployment weights. We had chosen a stable sedimentary environment ca. 20 m away from the hotspot confirmed earlier by online T lance measurements from the ship. The ROV then positioned the sensors across the area of interest (Fig. sensor cables LOOME), from the temperature hotspot towards the edge of the most recent mud flow (close to the geographic center). Via CSLI the functioning of COSTOF, the T-sensors and the camera AIM was recorded and final program optimizations were made. The deployment was a complex operation that went fully according to plan. The CSLI was also used to optimally position the camera, which has been placed facing a bubble stream and abundant fauna (Fig. Ifremer camera). Also there first temperature data were obtained. Recovery of LOOME, OBS and the T-lance will occur in autumn 2010, by a cruise with the FS Merian. The deployment of the long P- and T-lances was done by winch, and recovery was aided by the ROV, to hook on the ships winch.
On the Hakon Mosby we encountered the RV Jan Mayen, with our LOOME partner Jürgen Mienert as chief scientist, who recovered and redeployed the ocean bottom seismometer (OBS). The OBS was redeployed at the ideal position, at the edge of the most active area.
Deployment took place during the ARK-XXIV/2 FS Polarstern cruise (cruise leader M. Klages) in July 2009 using the ROV QUEST4000 of MARUM. LOOME was lowered to the seafloor by winch, and accurately positioned by the ROV Quest after the release of its deployment weights. We had chosen a stable sedimentary environment ca. 20 m away from the hotspot confirmed earlier by online T lance measurements from the ship. The ROV then positioned the sensors across the area of interest (Fig. sensor cables LOOME), from the temperature hotspot towards the edge of the most recent mud flow (close to the geographic center). Via CSLI the functioning of COSTOF, the T-sensors and the camera AIM was recorded and final program optimizations were made. The deployment was a complex operation that went fully according to plan. The CSLI was also used to optimally position the camera, which has been placed facing a bubble stream and abundant fauna (Fig. Ifremer camera). Also there first temperature data were obtained. Recovery of LOOME, OBS and the T-lance will occur in autumn 2010, by a cruise with the FS Merian. The deployment of the long P- and T-lances was done by winch, and recovery was aided by the ROV, to hook on the ships winch.
On the Hakon Mosby we encountered the RV Jan Mayen, with our LOOME partner Jürgen Mienert as chief scientist, who recovered and redeployed the ocean bottom seismometer (OBS). The OBS was redeployed at the ideal position, at the edge of the most active area.
Fotos of deployment, UW photos of LOOME made by ROV Quest, MARUM (Bremen, Germany).
Further literature:
1. Boetius, A., K. Ravenschlag, C. Schubert, D. Rickert, F. Widdel, A. Gieseke, R. Amann, B. B. Jørgensen, U. Witte, and O. Pfannkuche. 2000. A marine microbial consortium apparently mediating anaerobic oxidation of methane. Nature 407:623-626.
2. de Beer, D., E. Sauter, H. Niemann, N. Kaul, J. P. Foucher, U. Witte, M. Schlüter, and A. Boetius. 2006. In situ fluxes and zonation of microbial activity in surface sediments of the Håkon Mosby Mud Volcano. Limnol. Oceanogr. 51:1315-1331.
3. Elvert, M., E. C. Hopmans, T. Treude, A. Boetius, and E. Suess. 2005. Spatial variations of methanotrophic consortia at cold methane seeps: imnplications from a high-resolution molecular and isotopic approach. Geobiology 3:195-209.
4. Martens, C. S., and J. V. Klump. 1980. Biogeochemical cycling in an organic-rich coastal marine basin. I. Methane sediment-water exchange processes. Geoch.Cosmoc.Acta 44:471-490.
5. Michaelis, W., R. Seifert, K. Nauhaus, T. Treude, V. Thiel, M. Blumenberg, K. Knittel, A. Gieseke, K. Peterknecht, T. Pape, A. Boetius, R. Amann, B. B. Jørgensen, F. Widdel, J. Peckmann, N. V. Pimenov, and M. B. Gulin. 2002. Microbial reefs in the Black Sea fueled by anaerobic oxidation of methane. Science 297:1013-1015.
6. Treude, T., M. Krüger, A. Boetius, and B. B. Jørgensen. 2005. Environmental control on anaerobic oxidation of methane in the gassy sediments of Eckenfoerde Bay (German Baltic). Limnol. Oceanogr. 50:1771-1786.
7. Blandin, J., and J.F. Rolin. 2005.An Array of Sensors for the Seabed Monitoring of Geohazards, a Versatile Solution for the Long -Term Real-Time Monitoring of Distributed Seabed Parameters. Sea Technology, Volume 46, No. 12.
8. Blandin J., A. Vangriesheim, J. Legrand, J.Y. Coail, B. Leildé. 2007. Real time transmission of current and turbidity data from the near bottom Var canyon system. Martech 2007 Second International Workshop on Marine Technology proceedings.
1. Boetius, A., K. Ravenschlag, C. Schubert, D. Rickert, F. Widdel, A. Gieseke, R. Amann, B. B. Jørgensen, U. Witte, and O. Pfannkuche. 2000. A marine microbial consortium apparently mediating anaerobic oxidation of methane. Nature 407:623-626.
2. de Beer, D., E. Sauter, H. Niemann, N. Kaul, J. P. Foucher, U. Witte, M. Schlüter, and A. Boetius. 2006. In situ fluxes and zonation of microbial activity in surface sediments of the Håkon Mosby Mud Volcano. Limnol. Oceanogr. 51:1315-1331.
3. Elvert, M., E. C. Hopmans, T. Treude, A. Boetius, and E. Suess. 2005. Spatial variations of methanotrophic consortia at cold methane seeps: imnplications from a high-resolution molecular and isotopic approach. Geobiology 3:195-209.
4. Martens, C. S., and J. V. Klump. 1980. Biogeochemical cycling in an organic-rich coastal marine basin. I. Methane sediment-water exchange processes. Geoch.Cosmoc.Acta 44:471-490.
5. Michaelis, W., R. Seifert, K. Nauhaus, T. Treude, V. Thiel, M. Blumenberg, K. Knittel, A. Gieseke, K. Peterknecht, T. Pape, A. Boetius, R. Amann, B. B. Jørgensen, F. Widdel, J. Peckmann, N. V. Pimenov, and M. B. Gulin. 2002. Microbial reefs in the Black Sea fueled by anaerobic oxidation of methane. Science 297:1013-1015.
6. Treude, T., M. Krüger, A. Boetius, and B. B. Jørgensen. 2005. Environmental control on anaerobic oxidation of methane in the gassy sediments of Eckenfoerde Bay (German Baltic). Limnol. Oceanogr. 50:1771-1786.
7. Blandin, J., and J.F. Rolin. 2005.An Array of Sensors for the Seabed Monitoring of Geohazards, a Versatile Solution for the Long -Term Real-Time Monitoring of Distributed Seabed Parameters. Sea Technology, Volume 46, No. 12.
8. Blandin J., A. Vangriesheim, J. Legrand, J.Y. Coail, B. Leildé. 2007. Real time transmission of current and turbidity data from the near bottom Var canyon system. Martech 2007 Second International Workshop on Marine Technology proceedings.
Loome crew members on the Polarstern
Dirk de Beer, Jerome Blandin, Antje Boetius, Tomas Feseker, Jean Paul Foucher, Julien LeGrand, Jürgen Mienert, Francois Harmegnies, Karine Olu, Volker Ratmeyer, Vitali Schkel, Marc Viehweger, Christoph Waldmann, Frank Wenzhoefer, Gero Wetzel
Dirk de Beer, Jerome Blandin, Antje Boetius, Tomas Feseker, Jean Paul Foucher, Julien LeGrand, Jürgen Mienert, Francois Harmegnies, Karine Olu, Volker Ratmeyer, Vitali Schkel, Marc Viehweger, Christoph Waldmann, Frank Wenzhoefer, Gero Wetzel