- Departments
- Max Planck Research Group Microbial Metabolism
Max Planck Research Group Microbial Metabolism
Head of Group
MPI for Marine Microbiology
Celsiusstr. 1
D-28359 Bremen
Germany
Phone: |
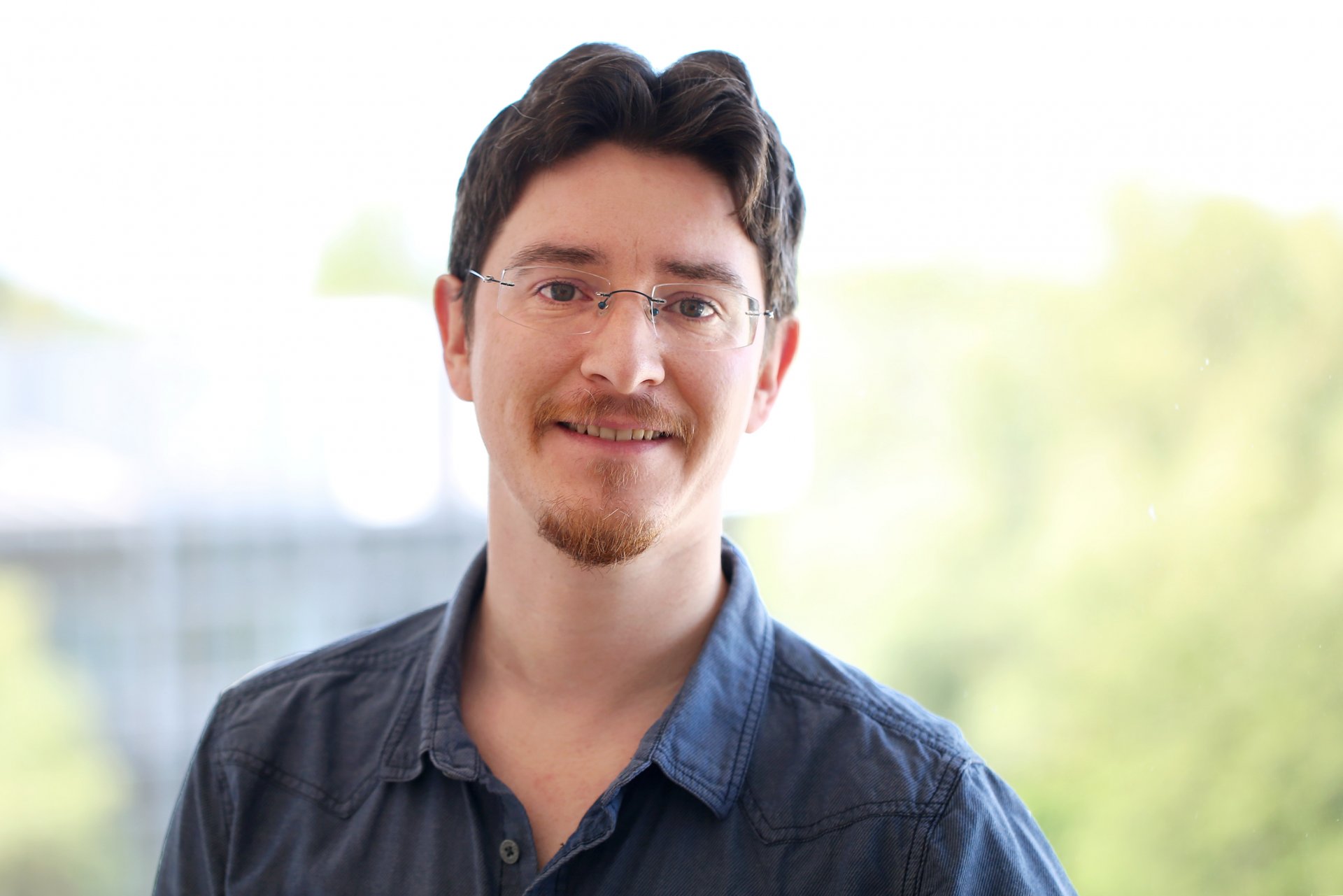
About our research
Some microbes, called methanogens, have the ability to produce methane, a greenhouse gas as well as a potent biofuel. Methanogens do not breathe oxygen. Instead, they derive all their energy from methane production through a process called methanogenesis, which provides 60 times less chemical energy (ATP) than oxygen respiration. Since methanogenesis is completely deactivated in presence of oxygen, these microorganisms are constrained to live in anaerobic environment with an extreme restriction of chemical energy.
However, methanogenesis is an extremely efficient process and the different chemical reactions involved in methane generation have been optimized during billions of years of evolution. More interestingly, some of the methanogens are complete chemoautotrophs: they have to build all their elementary bricks (DNA, RNA, protein, lipids, vitamins…) only from minerals and gases. With all these particularities, the study of methanogens is an open door to the past and future: they could have been one of the first life forms on our planet because of their “chemoautotrophic ancient metabolism” and nowadays, they could be used for new biotechnologies to efficiently capture carbon dioxide and produce methane.
The Microbial Metabolism Group aims to understand, at the molecular level, how methanogens are surviving and growing in extreme environments. How do they generate methane from different sources of carbon so efficiently? How do they convert minerals into the elementary bricks of life? And how do they protect themselves against stresses from their natural environment?
To find an answer to these questions, we must cultivate these microorganisms and study the different chemical reactions occurring inside them. The enzymes involved in the conversion of minerals and gases are proteins that orchestrate strange reactions highly challenging for chemists. We have to extract these enzymes, and sort them out from other proteins by using the native purification. We then pierce the molecular secret of their reaction by looking at them with X-ray crystallography, which means that we have to crystallize the enzymes, and use X-ray to get their pictures.
Understanding the “treasure chest” reactions of methanogens growing with so little energy is primordial if we want to transfer their secrets for synthetic chemistry. One of our focuses is to decipher their ability to fix nitrogen very efficiently. Nitrogen fixation, providing us with fertilizers and biofuel, consumes 3 percent of our worldwide energy (Haber-Bosch-Process). If we decipher how methanogens are dealing with this reaction, we could generate bio-mimic catalysts to improve the Haber-Bosch-Process.
During their first collaboration, Tristan Wagner and his collaborators from the Max Planck Institute for Marine Microbiology realized that they make a great match by joining the isolation of new microorganisms to the structural biology of their enzymes. As a biological model, Wagner uses Methanothermococcus thermolithotrophicus, a marine methanogen. The most critical part of his groups’ study is methanogen cultivation: we need special gases, equipments and most importantly the knowledge to take care of them.
The MPIMM gathers all these precious technical capabilities and the necessary know-how. This institute is at the first stage to discover new organisms from the deep ocean, a terra incognita where a lot of discoveries are waiting for us. This is a gold nugget for biochemists like me, which are looking for new metabolisms and new enzymes from freshly characterized microorganisms. As a structural biologist, I am highly excited to interact with the other teams of the MPIMM, to look at the metabolic pathways they work with and look for unforeseen enzymes. On the other hand, I also hope that this institute will help to widen my perspective, to zoom out of the atomic world and get a global picture at the ecosystem scale and to understand the interactions between them and our planet.